Define air navigation. Air navigation synonyms, air navigation pronunciation, air navigation translation, English dictionary definition of air navigation. English: air navigation n navigazione f aerea. Best small suvs all makes models. Italian / Italiano: aeronavigazione. The Future Air Navigation System (FANS) is an avionics system which provides direct data link communication between the pilot and the air traffic controller.The communications include air traffic control clearances, pilot requests and position reporting.
air navigation,
science and technology of determining the position of an aircraft with respect to the surface of the earth and accurately maintaining a desired course (see navigationnavigation,science and technology of finding the position and directing the course of vessels and aircraft. Early Navigational Techniques
In ancient times, mariners navigated by the guidance of the sun and stars and landmarks along the coast.
...Click the link for more information.).
Visual and Instrument Flight
The simplest and least sophisticated way to keep track of position, course, and speed is to use pilotage, a method in which landmarks are noted and compared with an aeronautical chart. Whether these landmarks are observed visually or on radar, this technique of air navigation is usually called flying under visual flight regulations (VFR). These establish the minimum weather conditions under which pilotage is permissible.
Pilotage is not satisfactory for long trips, especially over water or terrain lacking distinctive features. In these cases, or when weather conditions do not permit navigation by visual reference, planes must fly according to instrument flight regulations (IFR), which require that the aircraft be equipped with the necessary position-finding instruments and that the pilot be trained in operating those instruments. Also required under IFR is the filing of a flight plan with air traffic controlair traffic control,
the system by which airplanes are safely routed into and out of major airports. Air traffic control in the United States is centered in a number of regional control centers that route airplanes along established airways to airport traffic control centers.
...Click the link for more information. authorities at the departure point. The aircraft is then cleared for a given course and a given altitude. Air traffic controllers monitor the craft until it reaches its destination.
Aircraft Instruments
Light aircraft, flown by pilotage, typically have a simple set of navigational instruments, including an airspeed indicator (see pitot static systempitot static system
, device for measuring the rate at which a fluid flows. Among the principal applications of the device are an airspeed indicator for aircraft and a distance and speed indicator for ships.
...Click the link for more information.), an aneroid altimeter, and a magnetic compass. For supersonic and hypersonic aircraft the airspeed indicator is altered to show the airspeed as a Mach numberMach number
[for E. Mach], ratio between the speed of an object and the speed of sound in the medium in which the object is traveling. An airplane that has the velocity of Mach 3.0 is traveling at three times the speed of sound as measured in the prevailing atmospheric conditions.
...Click the link for more information., which is the ratio of the speed of an aircraft to the speed of sound. Advanced aircraft also use electronic systems to give the pilot highly accurate positional information for use during landing. The Instrument Landing System enables an airplane to navigate through clouds or darkness to an airport's runway; the Microwave Landing System, installed in U.S. airports beginning in 1988, is capable of landing the plane automatically, although the pilot always has the option of overriding manually.
Other navigational aids include the radio altimeteraltimeter
, device for measuring altitude. The most common type is an aneroid barometer calibrated to show the drop in atmospheric pressure in terms of linear elevation as an airplane, balloon, or mountain climber rises.
...Click the link for more information., a radar device that indicates the distance of the plane from the ground; the ground-speed indicator, which operates by measuring the Doppler shift in a radio wave reflected from the ground; and, in commercial airliners, the flight management computer, which can display altitude, speed, course, wind conditions, and route information, as well as monitor the airplane's progress through the airway. Other similar systems use inertial devices such as free-swinging pendulums and gyroscopes as references in determining position. These automated and semiautomated procedures free the pilot from many of the activities previously necessary for navigation and thus allow the pilot to concentrate on actually flying the aircraft. Another device which is useful in this way is the automatic pilot, which interprets data on direction, speed, attitude, and altitude to maintain an aircraft in straight, level flight on a given course at a given speed.
Airways and Radio Ranges
Basic to air traffic control are special air routes called airways. Airways are defined on charts and are provided with radio rangesradio range,
geographically fixed radio transmitter that radiates coded signals in all directions to enable aircraft and ships to determine their bearings. An aircraft or ship can determine its line of position and drift if it knows its bearing relative to the radio transmitter
...Click the link for more information., devices that allow the pilot whose craft has a suitable receiver to determine the plane's bearing and distance from a fixed location. The most common beacon is a very high frequency omnidirectional radio beacon, which emits a signal that varies according to the direction in which it is transmitted. Using a special receiver, an air navigator can obtain an accurate bearing on the transmitter and, using distance-measuring equipment (DME), distance from it as well.
The system of radio ranges around the United States is often called the VORTAC system. For long distances other electronic navigation systems have been developed: Omega, accurate to about two miles (3 km); Loran-C, accurate to within .25 mi (.4 km) but available only in the United States; and the Global Positioning System (GPS), a network of 24 satellites that is accurate to within a few yards and is making radio ranging obsolete.
Bibliography
See J. Elliott and G. Guerny, Pilot's Handbook of Navigation (1977).
Navigation, Air
the science of the methods and equipment for guiding aircraft (airplanes, helicopters, missiles, and so on); the aggregate of operations used by ground control or on-board facilities to determine navigational elements, and also their use to guide the aircraft. The principles of air navigation originated in sea navigation, which was developed in ancient times. In particular, the method of the magnetic compass and nautical astronomy were borrowed from it.
Air navigation ensures that an aircraft is guided along a trajectory determined by the flight route and profile, according to a prescribed plan that regulates the flight conditions from takeoff to landing at a prescribed time. In addition, air navigation solves particular navigation problems, such as the maintenance of prescribed distances and time intervals between aircraft on routes with dense air traffic or upon leaving a route for a landing approach, the prevention of collisions of airplanes with terrain obstacles (such as mountains), and the convergence of two aircraft in flight (for example, a rendezvous with a tanker for refueling). When a flight is carried out according to a predetermined route and plan, the task of air navigation, in contrast to pilotage, reduces primarily to the acquisition of continuous or periodic information on the current navigational elements of the translational motion of the center of mass of the aircraft with respect to a coordinate system correlated to the surface of the earth.
Various types of equipment are used to determine the navigational elements (including course, drift angle, course angle, airspeed and ground speed, altitude, and the coordinates of the aircraft’s location). The equipment is divided into four main groups, according to the primary source of navigational information as follows.
(1) Geotechnical devices, which make possible determination of the relative altitude of flight, the magnetic course, and the location of the aircraft by measuring various parameters of the earth’s geophysical fields (such as the magnetic and gravitational fields). Such equipment includes altimeters, devices for measuring airspeed and ground speed, magnetic and gyromagnetic compasses, directional gyroscopes, optical sights, and inertial navigation systems.
(2) Radio aids, which make possible determination of the true altitude, ground speed, and location of an aircraft by measuring various parameters of the electromagnetic field on the basis of radio signals from special transmitters. Such aids include radio altimeters, radio beacons, radio compasses, and radio navigation systems.
(3) Astronomical equipment, which makes possible determination of the course and location of an aircraft. This includes astrocompasses, sextants, and stellar trackers.
(4) Illumination devices, which are intended to facilitate the landing of aircraft under complex weather conditions and at night, and also to facilitate orientation (beacons).
Since advantages and shortcomings are inherent in each group of technical navigational aids, aids that use various principles are combined as sensors to form unified integrated systems to ensure the accurate flight of an aircraft along a prescribed route under any weather conditions. In such systems the main navigation problems are solved by means of analog or digital computers, and a flight plan is drawn up (including such factors as the coordinates of the points on the route, the altitudes and speeds of flyover of points, and the coordinates of radio navigation systems). Integrated navigation systems that are linked to an autopilot can accomplish automatic flight over the entire route, and also a landing approach, even when the surface of the earth cannot be seen. The integrated navigation system usually determines the position of the aircraft on the basis of three coordinates: two are the projections of the aircraft’s center of mass onto a horizontal plane (longitude and latitude), and one is the altitude. To orient an aircraft it is sufficient to know the two coordinates in the horizontal plane. The flight route is monitored on the basis of the route line, which is determined by a projection of the ground speed vector. This vector is found by adding the measured vectors of airspeed (the speed of the aircraft with respect to the air) and the speed of movement of the air with respect to the surface of the earth. The altitude is measured with an altimeter.
Various methods are used to determine the instantaneous coordinates of the aircraft’s position in flight. The three main methods are as follows: (1) dead reckoning, which is based on determination of the lines (surfaces) of the aircraft’s position by discrete or continuous summation of its measured velocity or acceleration over time; (2) the positional method (position lines), which is used for direct determination of the position lines (surfaces) of an aircraft without taking into consideration the distance it has traveled, by finding the coordinates of the aircraft’s position with respect to known ground reference points or heavenly bodies; (3) the comparative scanning method (orientation), which is used to determine an aircraft’s location either by comparing the actually observed picture of the terrain according to identified ground reference points (including visual, radar, and magnetic references) with a geographic map or standard model of the terrain or by comparing a section of the sky with a star chart. The specific characteristics of the guidance of various types of aircraft, and also the class and function of the aircraft, the regions where the craft are used, and the nature of the route, determine the composition of integrated air navigation systems. The equipment and methods to be used in air navigation are selected in accordance with a plan drawn up in advance by the navigator.
The necessity of ensuring the greatest possible safety of air traffic in spite of its increasing density, the growth in the number and length of air routes, and the further increase in the flying speeds of aircraft has led to the development and introduction of automatic integrated systems for air navigation and air traffic control.
REFERENCES
Spravochnik aviatsionnogo shturmana. Edited by V. I. Sokolov. Moscow, 1957.Kirst, M. A. Navigatsionnaia kibernetika poleta. Moscow, 1971.
Air Navigation System
air navigation
[¦er ‚nav·ə′gā·shən] (navigation)Want to thank TFD for its existence? Tell a friend about us, add a link to this page, or visit the webmaster's page for free fun content.
Link to this page:
The Future Air Navigation System (FANS) is an avionics system which provides direct data link communication between the pilot and the air traffic controller. The communications include air traffic control clearances, pilot requests and position reporting.[1] In the FANS-B equipped Airbus A320 family aircraft, an Air Traffic Services Unit (ATSU) and a VHF Data Link radio (VDR3) in the avionics rack and two data link control and display units (DCDUs) in the cockpit enable the flight crew to read and answer the controller–pilot data link communications (CPDLC) messages received from the ground.[2]
Overview of FANS[edit]
The world's air traffic control system still uses components defined in the 1940s following the 1944 meeting in Chicago which launched the creation of the International Civil Aviation Organization (ICAO). This traditional ATC system uses analog radio systems for aircraft Communication, navigation and surveillance (CNS).
Air traffic control's ability to monitor aircraft was being rapidly outpaced by the growth of flight as a mode of travel. In an effort to improve aviation communication, navigation, surveillance, and air traffic management ICAO standards for a future system were created, this integrated system is known as the Future Air Navigation System (FANS) and allows controllers to play a more passive monitoring role through the use of increased automation and satellite based navigation.
In 1983, ICAO established the special committee on the Future Air Navigation System (FANS), charged with developing the operational concepts for the future of air traffic management (ATM). The FANS report was published in 1988 and laid the basis for the industry's future strategy for ATM through digital CNS using satellites and data links. Work then started on the development of the technical standards needed to realise the FANS Concept.
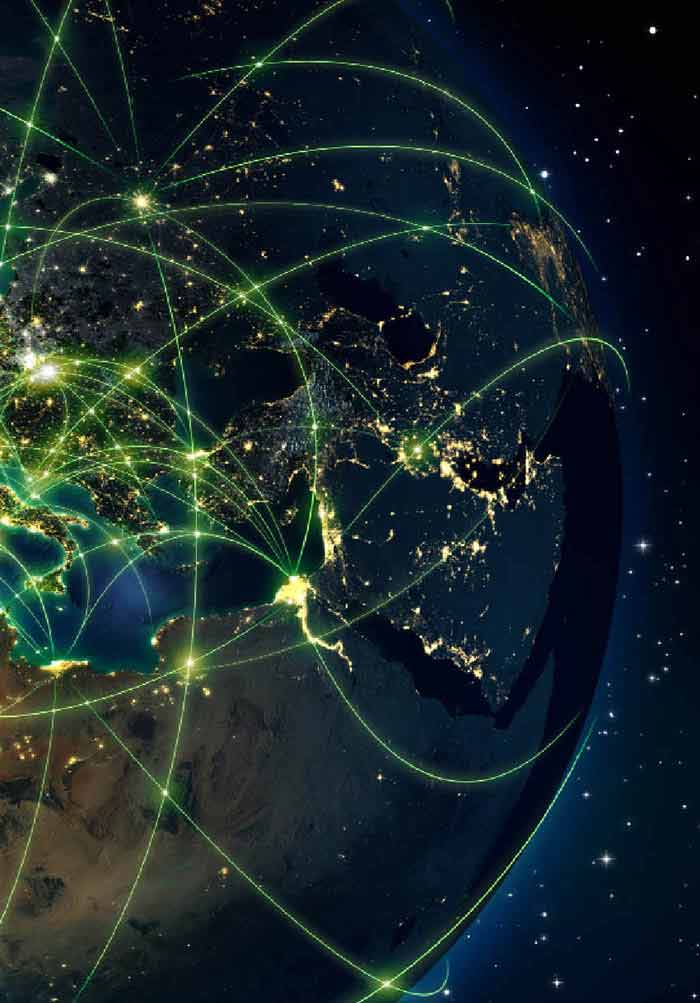
In the early 1990s, the Boeing Company announced a first generation FANS product known as FANS-1. This was based on the early ICAO technical work for automatic dependent surveillance (ADS) and controller–pilot data link communications (CPDLC), and implemented as a software package on the flight management computer of the Boeing 747-400. It used existing satellite based ACARS communications (Inmarsat the airplanes in the control area and uses VHF voice to provide instructions to the flight crews to ensure separation. Because the position of the aircraft is updated frequently and VHF voice contact timely, separation standards (the distance by which one aircraft must be separated from another) are less. This is because the air traffic controller can recognize problems and issue corrective directions to multiple airplanes in a timely fashion. Separation standards are what determine the number of airplanes which can occupy a certain volume of airspace.
Procedural control is used in areas (oceanic or land) which do not have radar. The FANS concept was developed to improve the safety and efficiency of airplanes operating under procedural control. This method uses time-based procedures to keep aircraft separated. The separation standard is determined by the accuracy of the reported positions, frequency of position reports, and timeliness of communication with respect to intervention. Non-FANS procedural separation uses Inertial Navigation Systems for position, flight crew voice reports of position (and time of next waypoint), and High Frequency radio for communication. The INS systems have error introduced by drifting after initial alignment. This error can approach 10 nmi (19 km).
HF radio communication involves contacting an HF operator who then transcribes the message and sends it to the appropriate ATC service provider. Responses from the ATC Service Provider go to the HF radio operator who contacts the airplane. The voice quality of the connection is often poor, leading to repeated messages. The HF radio operator can also be saturated with requests for communication. This leads to procedures which keep airplanes separated by as much as 100 nmi (190 km) laterally, 10 minutes in trail, and 4,000 ft (1,200 m) in altitude. These procedures reduce the number of airplanes which can operate in a given airspace. If market demand pushes airlines to operate at the same time on a given route, this can lead to airspace congestion, which is handled by delaying departures or separating the airplanes by altitude. The latter can lead to very inefficient operation due to longer flying times and increased fuel burn.
ATC using FANS[edit]
The FANS concept involves improvements to Communication, navigation and surveillance (CNS).
Communication improvements[edit]
This involved a transition from voice communications to digital communications. Specifically ACARS was used as the communication medium. This allowed other application improvements. An application was hosted on the airplane known as controller–pilot data link communications (CPDLC). This allows the flight crew to select from a menu of standard ATC communications, send the message, and receive a response. A peer application exists on the ground for the air traffic controller. They can select from a set of messages and send communications to the airplane. The flight crew will respond with a WILCO, STANDBY, or REJECT. The current standard for message delivery is under 60 seconds one way.
Navigation improvements[edit]
This involves a transition from inertial navigation to satellite navigation using the GPS satellites. This also introduced the concept of actual navigation performance (ANP). Previously, flight crews would be notified of the system being used to calculate the position (radios, or inertial systems alone). Because of the deterministic nature of the GPS satellites (constellation geometry), the navigation systems can calculate the worst case error based on the number of satellites tuned and the geometry of those satellites. (Note: it can also characterize the potential errors in other navigation modes as well). So, the improvement not only provides the airplane with a much more accurate position, it also provides an alert to the flight crew should the actual navigation performance not satisfy the required navigation performance (RNP).
Surveillance improvements[edit]
This involves the transition from voice reports (based on inertial position) to automatic digital reports. The application is known as ADS-C (automatic dependent surveillance, contract). In this system, an air traffic controller can set up a 'contract' (software arrangement) with the airplane's navigational system, to automatically send a position report on a specified periodic basis – every 5 minutes, for example. The controller can also set up a deviation contract, which would automatically send a position report if a certain lateral deviation was exceeded. These contracts are set up between ATC and the aircraft's systems, so that the flight crew has no workload associated with set-up.
FANS procedural control[edit]
The improvements to CNS allow new procedures which reduce the separation standards for FANS controlled airspace. In the South Pacific, they are targeting 30/30 (this is 30 nmi (56 km) lateral and 30 nmi (56 km) in trail). This makes a huge difference in airspace capacity.
History[edit]
ICAO[edit]
The International Civil Aviation Organization (ICAO) first developed the high level concepts starting with the initiation of the Special Committee on Future Air Navigation Systems in 1983. The final report was released in 1991 with a plan released in 1993.
Pacific engineering trials[edit]
FANS as we know it today had its beginning in 1991 with the Pacific Engineering Trials (PET). During these trials, airplanes installed applications in their ACARS units which would automatically report positions. These trials demonstrated the potential benefits to the airlines and airspace managers.
Implementation[edit]
United Airlines, Cathay Pacific, Qantas, and Air New Zealand approached the Boeing Company in 1993 and requested that Boeing support the development of a FANS capability for the 747-400 airplane. Boeing worked with the airlines to develop a standard which would control the interface between FANS-capable airplanes and air traffic service providers. The development of the FANS-capable aircraft systems proceeded simultaneously with the ATC ground system improvements necessary to make it work. These improvements were certified (using a QANTAS airplane) on June 20, 1995.
Both Boeing and Airbus continue to further develop their FANS implementations, Boeing on FANS-2 and Airbus on FANS-B. In the interim, Airbus came out with some enhancements to FANS-A, now referred to as FANS-A+. Various ground systems have been built, mainly by ATC organizations, to interoperate with FANS-1/A.
FANS interoperability team[edit]
The FANS interoperability team (FIT) was initiated in the South Pacific in 1998. The purpose of this team is to monitor the performance of the end-to-end system, identify problems, assign problems and assure they are solved. The members include airframe manufacturers, avionics suppliers, communication service providers, and air navigation service providers. Since this time, other regions have initiated FIT groups.
Service providers[edit]
Customers that operate aircraft need to get their FANS 1/A capable aircraft connected to both the ATN (Aeronautical Telecommunication Network) and to the Iridium and/or Inmarsat Satellite network. Commercial aircraft operators typically get their long haul fleet connected and have dedicated personnel to monitor and maintain the satellite and ground link while business aircraft and military aircraft operators contact companies like AirSatOne to commission the system for the first time, conduct functionality testing and to provide ongoing support. AirSatOne provide advanced FANS 1/A services through their Flight Deck Connect[3] portfolio of products. Flight Deck Connect includes a connection to the Iridium and/or Inmarsat satellites for FANS 1/A (via Datalink), and Safety Voice Services,[4] along with ancillary services (AFIS/ACARS) such as weather information, engine/airframe health and fault reports.
Operational approval[edit]
Some of the more advanced service providers such as AirSatOne and ARINC offer FANS 1/A testing services. When an aircraft is outfitted with FANS 1/A equipment either through the Type Certificate or STC process the equipment must demonstrate compliance with AC 20-140B for operational approval. As an example AirSatOne offers testing through the satellite and ATN network to support FANS 1/A functionality in accordance with RTCA DO-258A/ED-100A and provides test reports to meet the requirements of RTCA DO-258A/ED-100A, RTCA DO-306/ED-122 and FAA Advisory Circular AC 20-140B.[5] AirSatOne also provides first time system commissioning on each aircraft, troubleshooting testing and pre-flight maintenance checks to test FANS 1/A functionality either monthly or prior to flight in the FANS environment.
Milestones[edit]
On June 20, 1995, a QantasB747-400 (VH-OJQ) became the first aircraft to certify the Rolls-Royce FANS-1 package by remote type certification (RTC) in Sydney, Australia. It was followed by the first commercial flight from Sydney to Los Angeles on June 21. Subsequently, Air New Zealand certified the General Electric FANS-1 package, and United Airlines certified the Pratt & Whitney FANS-1 package.
Air Navigation Instruments
On May 24, 2004, a Boeing Business Jet completed the first North Atlantic flight by a business jet equipped with FANS. The airplane touched down at the European Business Aviation Convention and Exhibition (EBACE) in Geneva, Switzerland. The non-stop eight-hour, 4,000-nautical-mile (7,400 km) flight originating from Gary/Chicago International Airport in Gary, Indiana, was part of a North Atlantic Traffic trial conducted by the FANS Central Monitoring Agency (FCMA).
In August 2010, Aegean Airlines became the first airline to commit to upgrading its Airbus A320 fleet with a FANS-B+ retrofit system offered by Airbus.[6]
See also[edit]
- Aircraft Communications Addressing and Reporting System (ACARS)
- Aeronautical Telecommunication Network (ATN)
References[edit]
Air Navigation Charts
- ^An Assessment of Flight Crew Experiences with FANS-1 ATC Data Link
- ^de Cuendias, Sophie. 'The Future Air Navigation System, FANS B'. FAST 40. Airbus, an EADS Company (July, 2007): 13–19. ISSN1293-5476.
- ^'Flight Deck Connect™ by AirSatOne'. AirSatOne. Retrieved July 14, 2019.CS1 maint: discouraged parameter (link)
- ^'FAQ Inmarsat aircraft safety and communications' services'. Inmarsat. Retrieved July 14, 2019.CS1 maint: discouraged parameter (link)
- ^'AC 20-140B (Cancelled) - Guidelines for Design Approval of Aircraft Data Link Communication Systems Supporting Air Traffic Services (ATS) (Cancelled)'. Federal Aviation Administration. Retrieved July 14, 2019.CS1 maint: discouraged parameter (link)
- ^'Aegean commits to FANS-B+ upgrade for A320s'. ATW Online. August 16, 2010. Retrieved July 14, 2019.CS1 maint: discouraged parameter (link)
External links[edit]
Air Navigation Maps
